link to page 1 link to page 1 link to page 1 link to page 6 link to page 6 link to page 6
Pharmacological Research 120 (2017) 60–67
Contents lists available at
ScienceDirect
Pharmacological Research
j o u r n a l h o m e p a g e :
w w w . e l s e v i e r . c o m / l o c a t e / y p h r s
Review
Methamphetamine: Effects on the brain, gut and immune system
Monica D. Prakash, Kathy Tangalakis, Juliana Antonipillai, Lily Stojanovska,
Kulmira Nurgali
1, Vasso Apostolopoulos
∗,1
Immunology in Chronic Diseases Program, Centre for Chronic Disease, College of Health and Biomedicine, Victoria University, Melbourne, Australia
a r t i c l e i n f o
a b s t r a c t
Article history:
Methamphetamine (METH) is a powerful central nervous system stimulant which elevates mood, alert-
Received 10 November 2016
ness, energy levels and concentration in the short-term. However, chronic use and/or at higher doses
Received in revised form 8 February 2017
METH use often results in psychosis, depression, delusions and violent behavior. METH was formerly
Accepted 7 March 2017
used to treat conditions such as obesity and attention deficit hyperactivity disorder, but now is primarily
Available online 14 March 2017
used recreationally. Its addictive nature has led to METH abuse becoming a global problem. At a cellular
level, METH exerts a myriad of effects on the central and peripheral nervous systems, immune system
Keywords:
and the gastrointestinal system. Here we present how these effects might be linked and their potential
Anxiety
contribution to the pathogenesis of neuropsychiatric disorders. In the long term, this pathway could be
Depression
Ice
targeted therapeutically to protect people from the ill effects of METH use. This model of METH use may
Immune system
also provide insight into how gut, nervous and immune systems might break down in other conditions
Methamphetamine
that may also benefit from therapeutic intervention.
Nervous system
© 2017 Elsevier Ltd. All rights reserved.
Gastrointestinal system
Contents
1.
Introduction . . . . . . . . . . . . . . . . . . . . . . . . . . . . . . . . . . . . . . . . . . . . . . . . . . . . . . . . . . . . . . . . . . . . . . . . . . . . . . . . . . . . . . . . . . . . . . . . . . . . . . . . . . . . . . . . . . . . . . . . . . . . . . . . . . . . . . . . . . . . . 60
2.
METH and the blood-brain barrier . . . . . . . . . . . . . . . . . . . . . . . . . . . . . . . . . . . . . . . . . . . . . . . . . . . . . . . . . . . . . . . . . . . . . . . . . . . . . . . . . . . . . . . . . . . . . . . . . . . . . . . . . . . . . . . . . . . . . 61
3.
Neurological effects of METH . . . . . . . . . . . . . . . . . . . . . . . . . . . . . . . . . . . . . . . . . . . . . . . . . . . . . . . . . . . . . . . . . . . . . . . . . . . . . . . . . . . . . . . . . . . . . . . . . . . . . . . . . . . . . . . . . . . . . . . . . . . 61
4.
Sympathetic and parasympathetic regulation of the immune system . . . . . . . . . . . . . . . . . . . . . . . . . . . . . . . . . . . . . . . . . . . . . . . . . . . . . . . . . . . . . . . . . . . . . . . . . . . . . . . . 62
5.
The effects of METH on the gut-brain axis . . . . . . . . . . . . . . . . . . . . . . . . . . . . . . . . . . . . . . . . . . . . . . . . . . . . . . . . . . . . . . . . . . . . . . . . . . . . . . . . . . . . . . . . . . . . . . . . . . . . . . . . . . . . . 62
6.
METH and its effects on the immune system . . . . . . . . . . . . . . . . . . . . . . . . . . . . . . . . . . . . . . . . . . . . . . . . . . . . . . . . . . . . . . . . . . . . . . . . . . . . . . . . . . . . . . . . . . . . . . . . . . . . . . . . . . . 63
6.1.
The effects of METH on susceptibility to infection . . . . . . . . . . . . . . . . . . . . . . . . . . . . . . . . . . . . . . . . . . . . . . . . . . . . . . . . . . . . . . . . . . . . . . . . . . . . . . . . . . . . . . . . . . . . . . 64
6.2.
The effects of METH on inflammation and inflammatory markers . . . . . . . . . . . . . . . . . . . . . . . . . . . . . . . . . . . . . . . . . . . . . . . . . . . . . . . . . . . . . . . . . . . . . . . . . . . . . 64
6.3.
METH-associated immune cell changes . . . . . . . . . . . . . . . . . . . . . . . . . . . . . . . . . . . . . . . . . . . . . . . . . . . . . . . . . . . . . . . . . . . . . . . . . . . . . . . . . . . . . . . . . . . . . . . . . . . . . . . . 64
6.4.
The effects of METH on the expression of death receptor PD-1 and its ligand PD-L1 . . . . . . . . . . . . . . . . . . . . . . . . . . . . . . . . . . . . . . . . . . . . . . . . . . . . . . . . . . 65
7.
Conclusion and future prospects . . . . . . . . . . . . . . . . . . . . . . . . . . . . . . . . . . . . . . . . . . . . . . . . . . . . . . . . . . . . . . . . . . . . . . . . . . . . . . . . . . . . . . . . . . . . . . . . . . . . . . . . . . . . . . . . . . . . . . . 65
Acknowledgments . . . . . . . . . . . . . . . . . . . . . . . . . . . . . . . . . . . . . . . . . . . . . . . . . . . . . . . . . . . . . . . . . . . . . . . . . . . . . . . . . . . . . . . . . . . . . . . . . . . . . . . . . . . . . . . . . . . . . . . . . . . . . . . . . . . . . . 65
References . . . . . . . . . . . . . . . . . . . . . . . . . . . . . . . . . . . . . . . . . . . . . . . . . . . . . . . . . . . . . . . . . . . . . . . . . . . . . . . . . . . . . . . . . . . . . . . . . . . . . . . . . . . . . . . . . . . . . . . . . . . . . . . . . . . . . . . . . . . . . . 65
1. Introduction
euphoria
[1]. METH use is associated with severe neurological and
physical consequences (e.g. paranoia, violent behaviour, psychosis,
Methamphetamine (METH; also called crystal, chalk or ice) is
anxiety and depression) and has become a serious public health
an addictive stimulant that can be administered orally, smoked,
problem worldwide
[2,3].
snorted or injected. Smoking or intravenous injection delivers
METH was discovered in Japan in 1919 and was commercially
METH to the brain rapidly, resulting in immediate and intense
used in 1938 under the brand name Pervitin. It was especially pop-
ular for tired night-shift workers and was used during WWII by
Germany to treat fatigue in tired army troops
[4]. METH became
∗
widely available from 1943 to treat a range of disorders including
Corresponding author.
E-mail address:
[email address] (V. Apostolopoulos).
narcolepsy, depression, obesity, alcoholism and attention deficit
1 These authors contributed equally.
hyperactivity disorder (ADHD). As METH decreased appetite it was
http://dx.doi.org/10.1016/j.phrs.2017.03.009
1043-6618/© 2017 Elsevier Ltd. All rights reserved.
link to page 6 link to page 6 link to page 6 link to page 6 link to page 6 link to page 6 link to page 6 link to page 3 link to page 7 link to page 7 link to page 7 link to page 7 link to page 7 link to page 3 link to page 6 link to page 6 link to page 7 link to page 4 link to page 7 link to page 7 link to page 7 link to page 7 link to page 7 link to page 7 link to page 7 link to page 7 link to page 7 link to page 7 link to page 7 link to page 7 link to page 7 link to page 7 link to page 7 link to page 7
M.D. Prakash et al. / Pharmacological Research 120 (2017) 60–67
61
also well marketed to women for weight loss. Although prolonged
ates the METH-induced increase in BBB permeability, thus acting
METH use can cause severe neurological damage, prescribed METH
as a neuroprotective molecule
[32].
is still legally available under the brand name Desoxyn to treat
severe obesity, narcolepsy and ADHD
[5–7].
In recent years METH use has increased dramatically. In the USA,
approximately 1.3 million people over the age of 12 have reported
using METH. According to the 2011 United Nations survey, about
3. Neurological effects of METH
2.5% of Australians have tried METH, which is 3–5 times higher
than USA, Canada and UK (United Nations, 2011). In 2013, 7% of
The euphoric effects of METH occur due to release of the neu-
Australians over the age of 14 years reported having used METH,
rotransmitter dopamine, which is involved in the experience of
with 50% having used ice, the purest form of METH
[8].
pleasure, motivation and motor function. However, long-term use
Immediate effects of acute or short-term METH use include
of METH causes molecular changes in the dopamine system, con-
increased alertness, heart rate, blood pressure, body temperature
tributing to nerve terminal damage in the brain and leading to
and a loss of appetite. Long-term, regular METH use can lead
impaired motor skills, rapid cognitive decline, increased anxiety,
to severe tooth decay, infection, weight loss, malnutrition, kid-
psychotic disorders, violent behaviour, hallucination, delusions and
ney damage, liver damage, respiratory issues, paranoia, violent
depression
(Fig. 2) [33]. These brain changes persist for many years
behaviour, psychosis, severe anxiety and depression. Even when
after METH use has ceased
[34].
individuals stop taking METH, the symptoms may persist for many
Acute METH use causes an increase in neurotransmitter release,
years
[9–14].
leading to potential damage to the terminal ends of neurons and
METH has more potent effects in women than men. In fact,
ultimately alters brain function. A single high dose of METH causes
6-fold greater vulnerability to relapse of METH-seeking behavior
neurotoxicity to dopamine and serotonin producing neurons in
is evident in experimental female rats as compared to male rats
rodents
[35]. Positron emission tomography (PET) and magnetic
[15]. Changes in brain morphology, such as hippocampus volume
resonance spectroscopy (MRS) studies in abstinent METH users
reduction, were seen in METH-abstinent females but not in males
indicate a reduction of dopamine transporters (DAT)
[36,37] and
[16]. In addition, females that are undergoing treatment for METH
serotonin transporters (SERT)
[38,39] that lasts up to 3 years after
abuse have higher instances of psychological and physical trauma
cessation of METH use. Brain tissues from rodents exposed to METH
compared to males
[17].
and post-mortem brain tissues isolated from chronic METH users
Herein, we review the findings on METH-related neurolog-
demonstrate decreased levels of dopamine, serotonin, DAT and
ical and immunological effects, particularly neuro-immune cell
SERT in areas highly innervated by dopaminergic and serotonin-
stability, alteration of cytokine production, inflammation, immuno-
ergic axon terminals
[40].
suppression, signal transduction and gene regulation.
Gamma-aminobutyric acid (GABA) is a major inhibitory neuro-
transmitter in the brain. Disruption of inhibition via GABA receptors
can lead to dopamine and serotonin dysfunction and promote
depression, anxiety, stress and cognition. Similar reductions in
2. METH and the blood-brain barrier
neurotransmitters are also observed in a number of chronic neu-
rological disorders such as Parkinson’s and Alzheimer’s disease
METH increases blood brain barrier (BBB) permeability, induc-
[41–43].
ing damage by altering the structure of proteins that are involved
Trace amine-associated receptor 1 (TAAR1) is a G-protein cou-
in BBB stability in mice
[18]. BBB permeability is also affected
pled receptor expressed on astrocytes, lymphocytes and neurons
by body temperature, oxidative stress and inflammation, all of
and negatively regulates neurotransmission via dopamine, nore-
which are impacted by METH use (
Fig. 1). Both hyperthermia and
pinephrine and serotonin in the central nervous system (CNS)
hypothermia alter BBB permeability, although hypothermia has
[44–46]. It is an intracellular receptor predominantly found in the
less effect
[19]. Oxidative stress and excess inflammation is also
cytoplasm of presynaptic terminals and is poorly expressed on the
associated with BBB damage in a number of neurodegenerative
cell membrane
[45]. Activated TAAR1 reduces dopamine receptor
disorders
[20–24]. Recently, liquid chromatography-mass spec-
activity and increases cyclic adenosine monophosphate (cAMP),
trometry (LC–MS/MS) analysis of extracts from rat brains following
protein kinase A and protein kinase C activation. Subsequently,
METH exposure identified changes in 18 proteins (11 from the
DAT is phosphorylated, leading to inhibition of dopamine trans-
hippocampus and 7 in the olfactory bulb); 13 of which were upreg-
port
[47,48]. TAAR1 signaling also activates transcription factor
ulated and 5 were downregulated. The modified proteins were
cAMP response element-binding protein (CREB) and nuclear factor
predominantly involved in cell death, inflammation, oxidation and
of activated T-cell (NFAT), which are associated with immune cell
apoptotic pathways
[25]. In addition, alterations of endothelial cell
activation and proliferation
[49,50]. There are numerous studies
structure and function, with increased levels of ROS, are observed
that examine the effect of METH on TAAR1. METH directly activates
in METH-related BBB disruptions
[26,27].
TAAR1 in vitro and increases the intracellular cAMP levels in human
METH induces peripheral kidney and liver damage that leads
HEK-393 fibroblasts
[51]. TAAR1 mRNA expression in resting T cells
to toxic ammonia levels in the blood and subsequently, the brain.
increases in response to METH administration
[52]. METH increases
Ammonia that is not cleared by the liver as normal accumulates and
intracellular cAMP levels in human astrocytes whereas TAAR1
causes oxidative damage of endothelial cells, activation of matrix
knockout cells have significantly reduced cAMP levels in response
metalloproteases (MMPs) and neuro-inflammation via microglia
to METH administration
[53]. Interestingly, TAAR1 knockout mice
and astrocyte activation, leading to BBB disruption
[28–30] (Fig. 1).
show no significant difference in body weight, temperature, loco-
Furthermore, METH alters BBB permeability via dysregulation of
motor activity and other behaviours compared to wild-type mice;
tight junction proteins including occludin, claudin-5, and ZO family
however increased firing rate of dopaminergic and serotonin-
proteins
[18,26,27,31]. Cytoskeletal rearrangement is also per-
ergic neurons are noted
[54–56]. Conversely, TAAR1 transgenic
turbed, with increased actin polymerization and expression of
mice show increased sensitivity to METH. RO5203648, a selective
actin-binding protein Arp2/3 complex observed following METH
TAAR1 agonist, alleviates METH-induced neurochemical effects in
administration
[18]. Interestingly, galectin-1, which is highly
rats, including hyperactivity, psychomotor effects and addiction
expressed in endothelial cells involved in BBB remodeling, allevi-
[57–59].
link to page 7 link to page 7 link to page 7 link to page 7 link to page 7 link to page 8 link to page 4 link to page 8 link to page 8
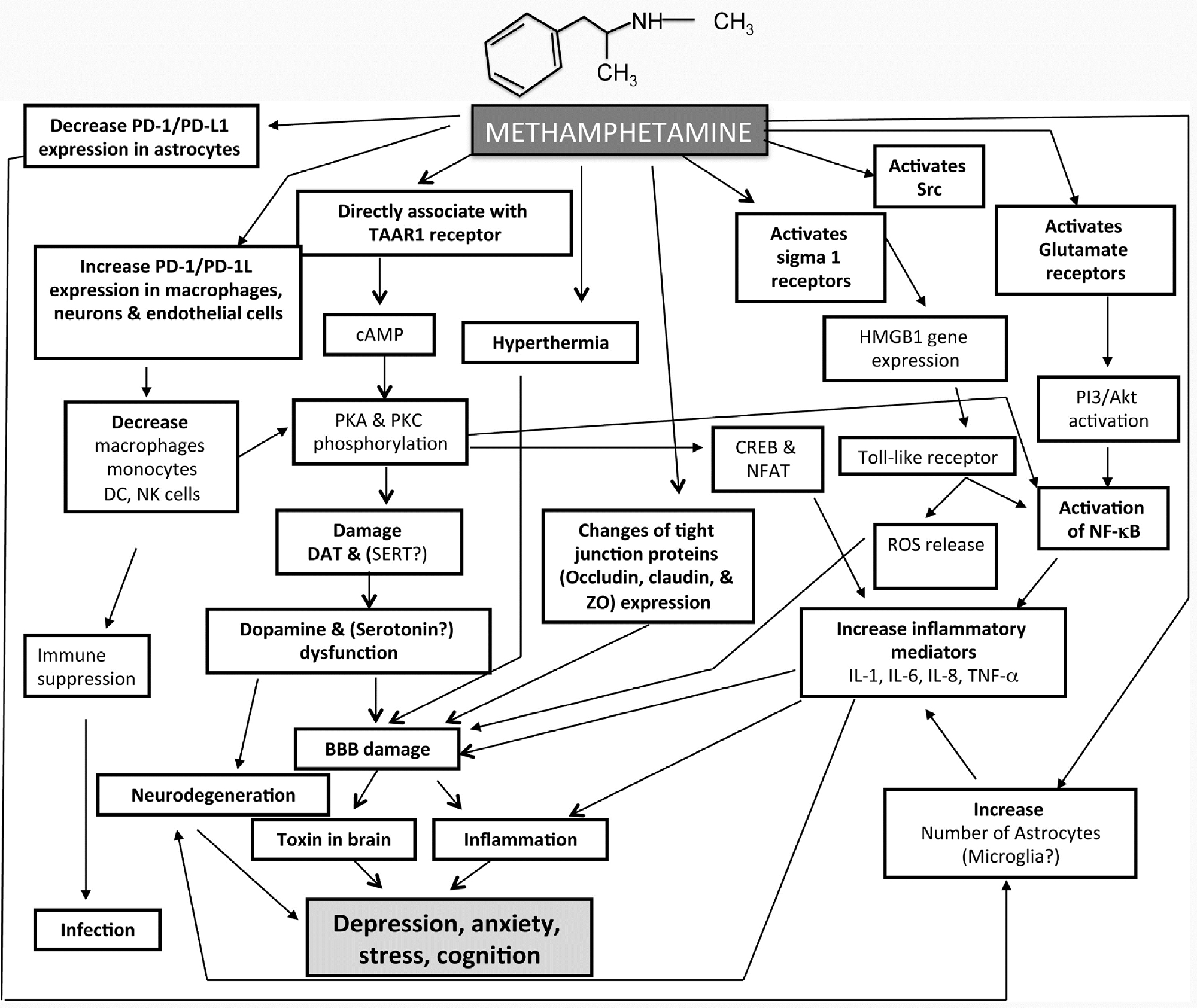
62
M.D. Prakash et al. / Pharmacological Research 120 (2017) 60–67
Fig. 1. Schematic diagram of the complex signals activated by METH.
4. Sympathetic and parasympathetic regulation of the
5. The effects of METH on the gut-brain axis
immune system
The rapid and sustained release of norepinephrine following
Sympathetic and parasympathetic nervous systems play an
METH use results in arterial vasoconstriction, leading to tachy-
important role in regulating the immune system. The sympathetic
cardia and hypertension. Similar effects can also be seen in the
nervous system is involved in stress-induced remodelling of lymph
mesenteric vessels of the gut, leading to acute intestinal ischemia
node innervation; increased norepinephrine and epinephrine
[69,70]. In METH users, the most common effects of gastrointesti-
levels inhibit immune cell functions and promote intestinal
nal (GI) vasoconstriction and bowel ischemia include abdominal
inflammation
[60–62]. The parasympathetic nervous system
or stomach cramping, severe constipation and/or diarrhoea and
has an anti-inflammatory role via activation of the choliner-
tissue dehydration. In some cases, loss of blood flow to GI mus-
gic anti-inflammatory pathway
[63,64]. Acetylcholine decreases
cles leads to severe, potentially fatal conditions such as paralytic
the production of pro-inflammatory cytokines such as TNF-␣ by
ileus (
Fig. 2) [71]. Potential consequences of paralytic ileus include
human macrophages through nicotinic receptors
[65]. Vagus nerve
severe infection, tissue death (gangrene), perforation of the intesti-
stimulation attenuates the systemic inflammatory response to
nal wall and serious disruptions in the levels of electrolytes. In
endotoxins and intestinal inflammation
[63,66]. The vagus nerve
severe cases, bowel infarction can lead to development of septic
also indirectly modulates immune activity of the spleen through
shock with multiple organ failure
[70].
connections with the splenic sympathetic nerve
[67,68]. However,
Bowel ischemia is associated with increased intestinal perme-
the effects of METH on the activity of sympathetic and vagus nerves
ability, oxidative and nitrosative stress. Several findings suggest
and their modulation of systemic and local immune responses have
that dysfunction of the intestinal mucosal barrier leading to
not been studied.
increased intestinal permeability plays an important role in the
pathophysiology of anxiety, stress, depression, cognitive decline,
link to page 3 link to page 8 link to page 8 link to page 8 link to page 8 link to page 8 link to page 8 link to page 8 link to page 4 link to page 8
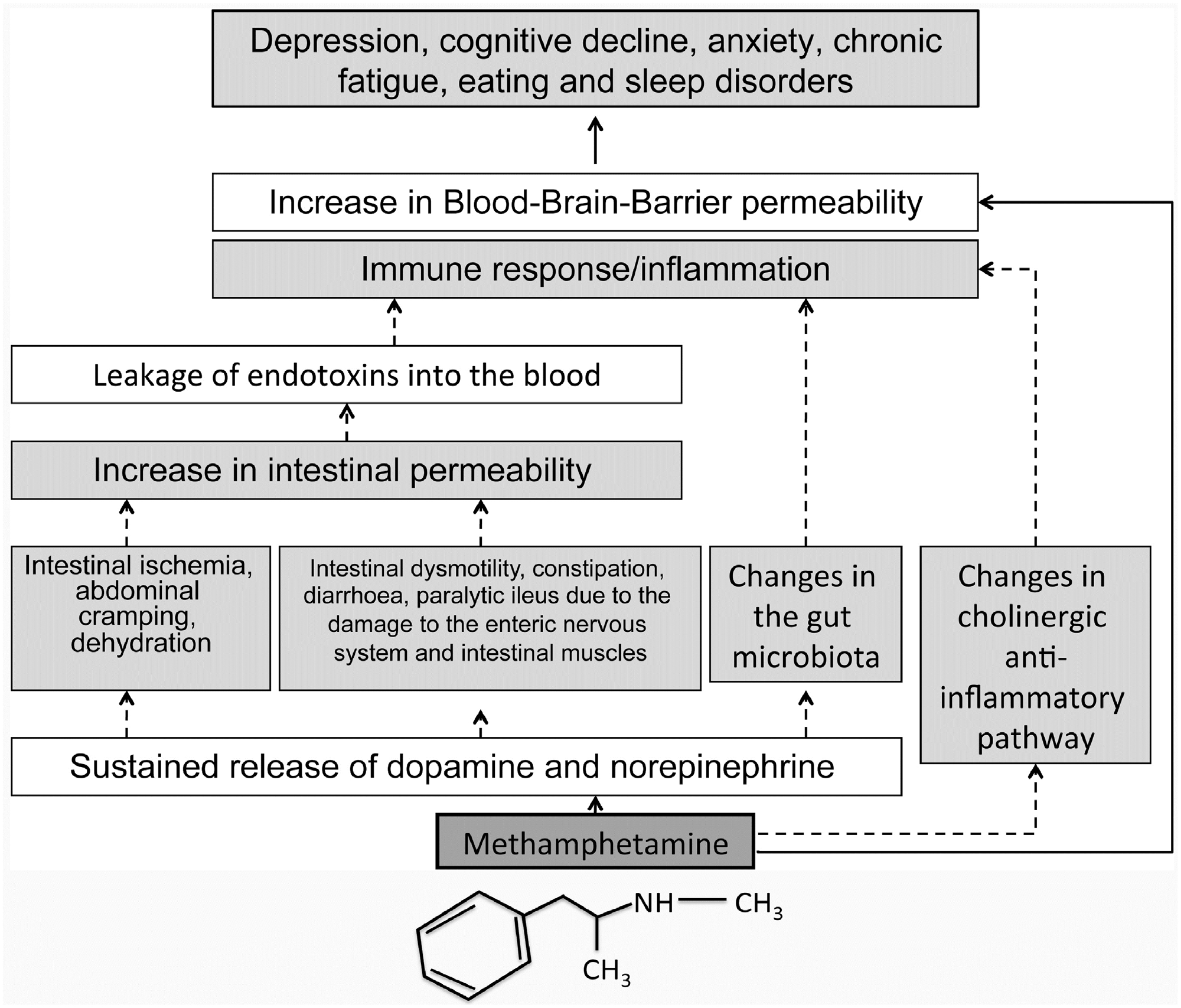
M.D. Prakash et al. / Pharmacological Research 120 (2017) 60–67
63
Fig. 2. METH (C10H15N) and the gut-brain axis. Solid arrows are known effects of METH and dotted arrows are hypothesised effects.
chronic fatigue and eating and sleep disorders. All of these are
tive nitrogen species (RNS) which can cause damage and death of
common in METH users
(Figs. 1, 2).
enteric neurons and subsequent GI dysfunction.
Disruption of the gut wall integrity, damage to intestinal epithe-
Recent advances in research have described the importance
lial cells and derangement of tight junctions leads to the leakage
of gut microbiota in many neuropsychiatric conditions including
of macromolecules, microbial products, and microbiota from the
autism, anxiety, depression, eating and sleep disorders. Current
intestinal lumen into the circulation, mesenteric lymph nodes,
evidence suggests that multiple mechanisms, including immune,
spleen and liver
[72]. With the concurrent increase in BBB perme-
endocrine and neuroendocrine pathways, may be involved in
ability following METH use, these gut-derived components have the
gut microbiota-to-brain signalling and that the brain can in turn
ability to enter the brain
[73]. Extensive release of dopamine and
alter microbial composition and behaviour via the enteric ner-
norepinephrine stimulates growth of bacteria which may also influ-
vous system
[79–81]. However, changes in the gut microbiota
ence neural activity in stress responsive brain areas
[74]. Therefore
after METH use and the interplay between intestinal microbiota,
the intestinal microbiota may act as a mediator in the communica-
immune response and neuropsychiatric manifestations associated
tion between the gut and the brain
[75]. However, the mechanisms
with METH use have not been studied
(Fig. 2)
underlying METH-induced increases in intestinal permeability and
damage to the GI tract leading to systemic immune response and
neuropsychiatric disorders are not clear.
Increases in intestinal permeability may be due to the inhibition
6. METH and its effects on the immune system
of GI motility observed in METH users
[71]. In the gut, dopamine
and norepinephrine act on receptors of the enteric nervous system
The human immune system has a profound influence on the
resulting in decreased bowel contractility, intestinal smooth mus-
brain. Increasing evidence shows that there are numerous interac-
cle tone and alteration of the migratory motor complex
[76–78].
tions between the nervous and immune systems
[82]. The immune
METH-mediated release of neurotransmitters might also lead to
system also plays an important role in the pathogenesis of neu-
generation of oxidative stress molecules, including ROS and reac-
ropsychiatric disorders including cognitive decline, anxiety, mood
changes and depressive states as well as increased attention,
link to page 8 link to page 5 link to page 8 link to page 6 link to page 8 link to page 8 link to page 8 link to page 6 link to page 8 link to page 8 link to page 8 link to page 8 link to page 8 link to page 8 link to page 8 link to page 8
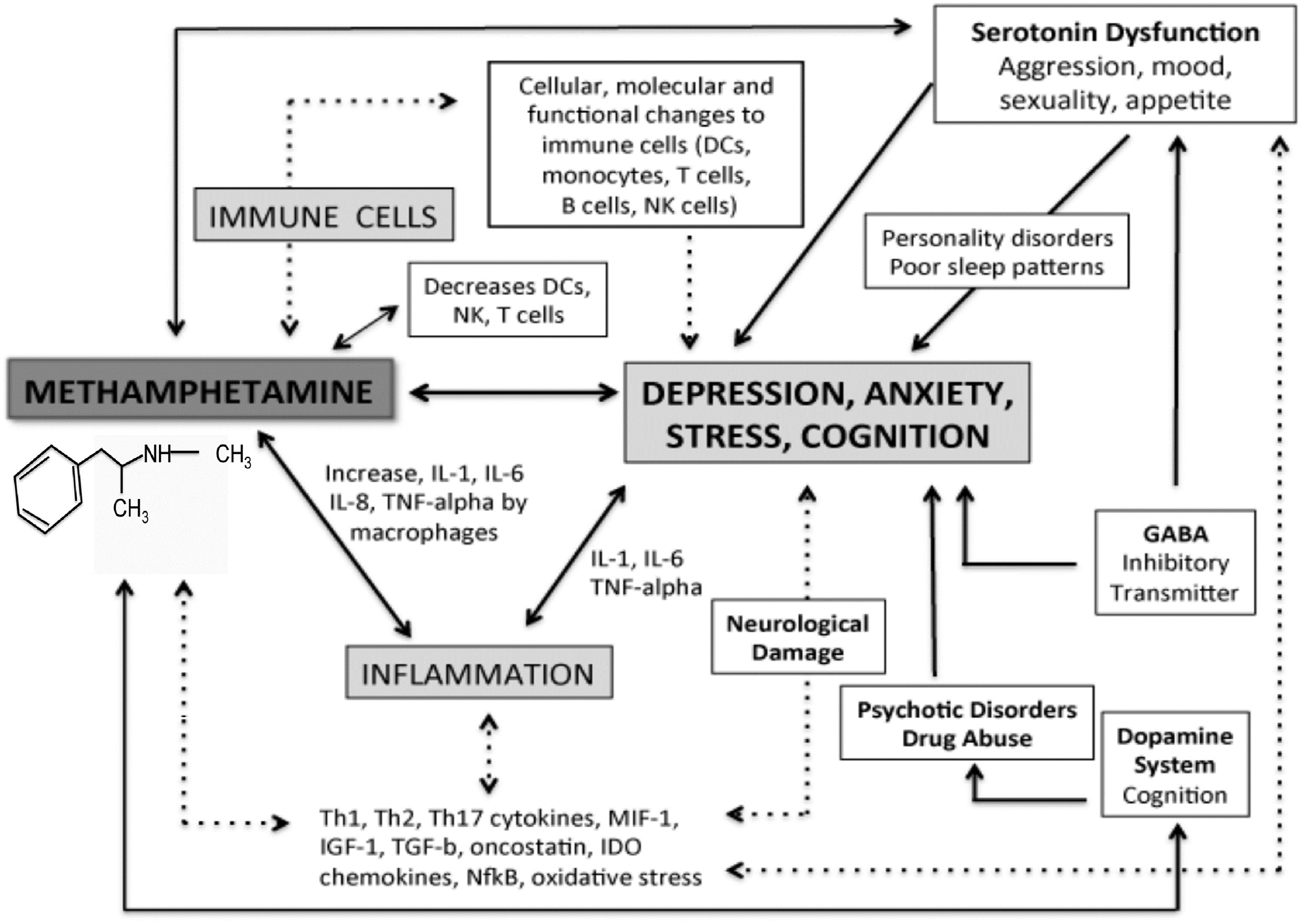
64
M.D. Prakash et al. / Pharmacological Research 120 (2017) 60–67
Fig. 3. Schematic diagram of the neuro-immunological affects of METH. Solid arrows are known effects of METH and dotted arrows are hypothesised effects.
decreased fatigue and euphoria rush
[80,83,84], which are asso-
related cell activation is seen in astrocytes and leads to excessive
ciated with METH use (
Fig. 3).
secretion of inflammatory cytokines such as IL-6 and IL-8 induc-
ing inflammation
[86,92], as well as enhancing expression of
6.1. The effects of METH on susceptibility to infection
chemokines and chemokine receptors such as CXCR4 and CCR5
in the brain
[93]. In addition, METH induces a pro-inflammatory
Chronic METH use and lack of hygiene leads to alteration in
profile of in vitro cultured macrophages, by upregulating TNF-␣,
primary physical barriers and increases the occurrence of skin
IL-8, CXCL16, CXCL1 and downregulating CCL7 (disrupting toll-like
infections
[85]. METH use also increases the risk of chronic infec-
receptor 9 (TLR-9) signaling pathway)
[94]. Suppression of TLR-9
tions such as methicillin-resistant Staphylococcus aureus (MRSA),
indicates that suppressed innate immune responses may ensue in
human immunodeficiency virus (HIV), hepatitis and sexually trans-
METH users.
mitted diseases
[3].
In the presence of METH, the number of macrophages, NK, DC,
6.3. METH-associated immune cell changes
monocytes and granulocytes are reduced, further contributing to
the increased susceptibility to infections
[86,87]. High METH dose
Immune cell mediated neuro-inflammation and neurodegener-
induces apoptotic death in rat thymic and splenic lymphocytes and
ation is induced by METH use. METH is a weak base and alkalizes
produces severe immunosuppression, which could also contribute
the acidic organelles within macrophages, leading to impaired
to the higher rate of infections observed in chronic METH users
phagocytosis, antigen processing and presentation
[95]. As a con-
[86,88]. METH also changes the cytokine response to retroviral
sequence, this can lead to a reduction of pathogen uptake and
infection in rodents
[89,90].
processing, increasing infections. Immunological factors such as
cytokines, chemokines and adhesion molecules are linked with
6.2. The effects of METH on inflammation and inflammatory
neuronal degeneration as well as neuropsychiatric complications
markers
[96,97].
METH modifies a number of immune cell (natural killer
Pro-inflammatory cytokines (IL-1, IL-6, IL-8, TNF-␣) have been
(NK) cells, dendritic cells (DC), monocytes, macrophages and
implicated in damaging and destroying existing neurons leading
granulocytes) activities, leading to immunosuppression
[86]. In
to the neurobiological manifestations of different mental states.
addition, METH affects antigen presenting cells (APCs) in the brain
Indeed, METH use results in IL-6 and IL-8 production by neuronal
(microglia and astrocytes) and leads to increased secretion of pro-
cells, leading to myelin degeneration in mice
[3]. Similarly, mice
inflammatory cytokines (IL-1, IL-6, IL-8), interferons and TNF-␣
treated with METH show increased expression of pro-inflammatory
[98]. Murine models show that METH modifies thymic and splenic
cytokines (IL-1) for up to 3 weeks in brain regions
[91]. METH-
cellularity, in turn altering peripheral T lymphocyte populations
link to page 8 link to page 8 link to page 8 link to page 8 link to page 8 link to page 8 link to page 8 link to page 8 link to page 8 link to page 8 link to page 8 link to page 8 link to page 8 link to page 8 link to page 8 link to page 8 link to page 8
M.D. Prakash et al. / Pharmacological Research 120 (2017) 60–67
65
[97]. Furthermore, METH suppresses adaptive immunity by altering
7. Conclusion and future prospects
T cell populations, specifically the CD4+/CD8+ T-cell ratio
[97,99].
Microglia and astrocytes usually perform compensatory actions
Recent advances in research have described the importance
during brain injury and protect the brain as excess neuro-
of gut microbiota in many neuropsychiatric conditions including
inflammation leads to damage. However, METH activates G-protein
autism, anxiety, depression, eating and sleep disorders. Current
receptors and initiates signalling of the Akt/NF-kB pathway
evidence suggests that multiple mechanisms, including immune,
to increase cell proliferation and cytokine secretion (IL-6 and
endocrine and neurocrine pathways, may be involved in gut-to-
IL-8) in astrocytes. The effect of METH-related IL-6 and IL-8
brain signalling and that the brain can in turn alter microbial
expression in astrocytes is reduced in the presence of 2-methyl-
composition and behaviour of the gut via the enteric nervous
6-(phenylethynyl)-pyridine (MPEP), an antagonist of metabotropic
system
[79–81]. Though it is apparent that METH use alters numer-
glutamate receptor 5 (mGlu5)
[100]. Furthermore, HIV-1 envelope
ous aspects of the nervous, immune and gastrointestinal systems,
protein gp120 acts synergistically with METH to further increase
changes in gut microbiota following METH use and the interplay
IL-6 expression
[101].
between intestinal microbiota, immune response and neuropsy-
METH activates the Sigma-1 receptor which in turn activates
chiatric manifestations associated with METH use are yet to be
NF-B via SRC/ERK, thus increasing high mobility group box-1
studied.
(HMGB1) gene expression in astrocytes
[102]. This promotes cell
proliferation and migration. HMGB1 also regulates gene transcrip-
Acknowledgments
tion and acts an inflammatory mediator by activating TLR-4
[103].
Activated immune cells such as macrophages and monocytes also
The authors would like to thank the Immunology of Chronic Dis-
increase the expression of HMGB1 during inflammation
[104,105].
eases Program, Centre for Chronic Disease, College of Health and
METH also increases glutamate release from a number of brain
Biomedicine, Victoria University for funding and helpful discus-
regions such as the striatum, cerebral cortex and hippocampus
sions. VA would like to thank Victoria University, College of Health
[106,107]. The phosphorylation of PI3/Akt molecules via glutamate
and Biomedicine, start up funds for funding the research.
receptor engagement leads to the activation of transcription factor
NF-B and ultimately facilitates inflammation, neurotoxicity and
References
apoptosis
[108]. Chronic METH exposure affects monoaminergic
neurons by the loss of DAT, SERT and vesicular monoamine trans-
[1]
P. Hauer, Systemic affects of methamphetamine use, S. D. Med. 63 (8) (2010)
porter type-2 (VMAT-2) in striatum and central gray matter of rat
285–287.
brain
[109].
[2]
N. Rommel, N.H. Rohleder, S. Wagenpfeil, R. Haertel-Petri, M.R. Kesting,
Evaluation of methamphetamine-associated socioeconomic status and
addictive behaviors, and their impact on oral health, Addict. Behav. 50
(2015) 182–187.
[3]
S.A. Salamanca, E.E. Sorrentino, J.D. Nosanchuk, L.R. Martinez, Impact of
6.4. The effects of METH on the expression of death receptor PD-1
methamphetamine on infection and immunity, Front. Neurosci. 8 (2014)
445.
and its ligand PD-L1
[4]
R.J. Defalque, A.J. Wright, Methamphetamine for Hitler’s Germany:
1937–1945, Bull. Anesth. Hist. 29 (2) (2011) 21–24, 32.
Programmed cell death-1 ligand (PD-L1), is a transmembrane
[5]
J. Cartier, D. Farabee, M.L. Prendergast, Methamphetamine use, self-reported
violent crime, and recidivism among offenders in California who abuse
protein that plays a major role in suppressing the immune sys-
substances, J. Interpers. Violence 21 (4) (2006) 435–445.
tem. T cells express the receptor PD-1 and upon interaction with
[6]
T. McGuinness, Methamphetamine abuse, Am. J. Nurs. 106 (12) (2006)
PD-L1 inhibitory signals are triggered resulting in T cell apopto-
54–59.
[7]
B.A. Rutkowski, California substance abuse research consortium, September
sis. Cancer cells that express high levels of PD-L1 as a mechanism
2005: update on recent methamphetamine trends in four California regions,
for immune evasion are associated with poor prognosis in patients
J. Psychoact. Drugs (Suppl. 3) (2006) 369–375.
[110–112]. In inflammatory disorders the expression of PD-L1 is
[8]
A. Roche, A. McEntee, J. Fischer, V. Kostadinov, Methamphetamine use in
Australia, in: National Centre for Education and Training on Addiction
reduced leading to activation of T cells. Thus, PD-L1 is important in
(NCETA), Flinders University, 2015.
regulating immune responses. The level of PD-L1 and PD-1 expres-
[9]
M.G. Kirkpatrick, M. Haney, S.K. Vosburg, S.D. Comer, R.W. Foltin, C.L. Hart,
sion in human brain cells under normal physiological conditions
Methamphetamine self-administration by humans subjected to abrupt shift
is low. However, activated neuro-immune cells such as astro-
and sleep schedule changes, Psychopharmacology (Berl.) 203 (4) (2009)
771–780.
cytes, microglia, T cells, B cells, macrophages, DC and non-immune
[10]
J. Mendelson, R. Rawson, T. Newton, G. Galloway, H. de Wit, S.L. Dewey, C.L.
cells (endothelial and epithelial cells) appear to have increased in
Hart, D.H. Epstein, Treatment of methamphetamine dependence, Mayo Clin.
expression
[113].
Proc. 83 (3) (2008) 369–370, author reply 370-361.
[11]
A.Y. Perez, M.G. Kirkpatrick, E.W. Gunderson, G. Marrone, R. Silver, R.W.
The expression of PD-L1 is elevated in brain endothelial cells, on
Foltin, C.L. Hart, Residual effects of intranasal methamphetamine on sleep,
macrophages and microglia, following METH exposure
[113]. PD-
mood, and performance, Drug Alcohol Depend. 94 (1–3) (2008) 258–262.
1 signalling attenuates phosphorylation of protein kinase C (PKC),
[12]
R. Padilla, A.V. Ritter, Meth mouth: methamphetamine and oral health, J.
Esthet. Restor. Dent. 20 (2) (2008) 148–149.
necessary for the activation of NF-kB and for production of IL-2
[13]
J.W. Shaner, N. Kimmes, T. Saini, P. Edwards, Meth mouth: rampant caries in
[114], thus METH exposure inhibits T cell activation. Overexpres-
methamphetamine abusers, Aids Patient Care STDS 20 (3) (2006) 146–150.
sion of PD-1 and PD-L1 following METH exposure in macrophages
[14]
N. Williams, J.S. Covington 3rd, Methamphetamine and meth mouth: an
overview, J Tenn Dent Assoc. 86 (4) (2006) 32–35.
may also suppress immunity by altering antigen presentation
[113].
[15]
J. Ruda-Kucerova, P. Amchova, Z. Babinska, L. Dusek, V. Micale, A. Sulcova,
Conversely, reductions in PD-1 and PD-L1 expression are noted
Sex differences in the reinstatement of methamphetamine seeking after
in astrocytes following METH exposure
[113]. Inhibition of PD-
forced abstinence in sprague-Dawley rats, Front. Psychiatry 6 (2015) 91.
[16]
J. Du, M. Quan, W. Zhuang, N. Zhong, H. Jiang, D.N. Kennedy, A. Harrington,
1/PD-L1 expression in astrocytes stimulates overproduction of
D. Ziedonis, X. Fan, M. Zhao, Hippocampal volume reduction in female but
inflammatory cytokines such as interleukins and leads to inflam-
not male recent abstinent methamphetamine users, Behav. Brain Res. 289
mation and neuronal damage. However, whether METH-related
(2015) 78–83.
reduction of PD-1/PD-L1 expression in astrocytes alters PKC acti-
[17]
Y.I. Hser, E. Evans, Y.C. Huang, Treatment outcomes among women and men
methamphetamine abusers in California, J. Subst. Abuse Treat. 28 (1) (2005)
vation or regulates the production of NFB and proinflammatory
77–85.
mediators such as IL-1, IL-6, IL-8 and TNF-␣ to cause the neuronal
[18]
M. Park, H.J. Kim, B. Lim, A. Wylegala, M. Toborek,
damage by inflammation are not clear and therefore warrants fur-
Methamphetamine-induced occludin endocytosis is mediated by the Arp2/3
complex-regulated actin rearrangement, J. Biol. Chem. 288 (46) (2013)
ther investigation.
33324–33334.
66
M.D. Prakash et al. / Pharmacological Research 120 (2017) 60–67
[19]
E.A. Kiyatkin, H.S. Sharma, Permeability of the blood-brain barrier depends
[43]
A.M. Murray, F.B. Weihmueller, J.F. Marshall, H.I. Hurtig, G.L. Gottleib, J.N.
on brain temperature, Neuroscience 161 (3) (2009) 926–939.
Joyce, Damage to dopamine systems differs between Parkinson’s disease and
[20]
X. Ji, W. Liu, K. Xie, W. Liu, Y. Qu, X. Chao, T. Chen, J. Zhou, Z. Fei, Beneficial
Alzheimer’s disease with parkinsonism, Ann. Neurol. 37 (3) (1995) 300–312.
effects of hydrogen gas in a rat model of traumatic brain injury via reducing
[44]
V.M. Lam, S. Espinoza, A.S. Gerasimov, R.R. Gainetdinov, A. Salahpour,
oxidative stress, Brain Res. 1354 (2010) 196–205.
In-vivo pharmacology of trace-amine associated receptor 1, Eur. J.
[21]
T.O. Price, N. Ercal, R. Nakaoke, W.A. Banks, HIV-1 viral proteins gp120 and
Pharmacol. 763 (Pt. B) (2015) 136–142.
Tat induce oxidative stress in brain endothelial cells, Brain Res. 1045 (1–2)
[45]
G.M. Miller, The emerging role of trace amine-associated receptor 1 in the
(2005) 57–63.
functional regulation of monoamine transporters and dopaminergic activity,
[22]
H.S. Sharma, A. Sharma, Nanoparticles aggravate heat stress induced
J. Neurochem. 116 (2) (2011) 164–176.
cognitive deficits, blood-brain barrier disruption, edema formation and
[46]
B. Borowsky, N. Adham, K.A. Jones, R. Raddatz, R. Artymyshyn, K.L. Ogozalek,
brain pathology, Prog. Brain Res. 162 (2007) 245–273.
M.M. Durkin, P.P. Lakhlani, J.A. Bonini, S. Pathirana, N. Boyle, X. Pu, E.
[23]
C.M. Zehendner, L. Librizzi, J. Hedrich, N.M. Bauer, E.A. Angamo, M. de Curtis,
Kouranova, H. Lichtblau, F.Y. Ochoa, T.A. Branchek, C. Gerald, Trace amines:
H.J. Luhmann, Moderate hypoxia followed by reoxygenation results in
identification of a family of mammalian G protein-coupled receptors, Proc.
blood-brain barrier breakdown via oxidative stress-dependent
Natl. Acad. Sci. U. S. A. 98 (16) (2001) 8966–8971.
tight-junction protein disruption, PLoS One 8 (12) (2013) e82823.
[47]
G.M. Miller, Avenues for the development of therapeutics that target trace
[24]
A.G. Kermode, A.J. Thompson, P. Tofts, D.G. MacManus, B.E. Kendall, D.P.
amine associated receptor 1 (TAAR1), J. Med. Chem. 55 (5) (2012)
Kingsley, I.F. Moseley, P. Rudge, W.I. McDonald, Breakdown of the
1809–1814.
blood-brain barrier precedes symptoms and other MRI signs of new lesions
[48]
J.J. Maguire, W.A. Parker, S.M. Foord, T.I. Bonner, R.R. Neubig, A.P. Davenport,
in multiple sclerosis. Pathogenetic and clinical implications, Brain 113 (Pt. 5)
International union of pharmacology: LXXII. Recommendations for trace
(1990) 1477–1489.
amine receptor nomenclature, Pharmacol. Rev. 61 (1) (2009) 1–8.
[25]
R. Zhu, T. Yang, F. Kobeissy, T.H. Mouhieddine, M. Raad, A. Nokkari, M.S.
[49]
H.N. Panas, L.J. Lynch, E.J. Vallender, Z. Xie, G.L. Chen, S.K. Lynn, T.S. Scanlan,
Gold, K.K. Wang, Y. Mechref, The effect of chronic methamphetamine
G.M. Miller, Normal thermoregulatory responses to 3-iodothyronamine,
exposure on the hippocampal and olfactory bulb neuroproteomes of rats,
trace amines and amphetamine-like psychostimulants in trace amine
PLoS One 11 (4) (2016) e0151034.
associated receptor 1 knockout mice, J. Neurosci. Res. 88 (9) (2010)
[26]
S.D. Mahajan, R. Aalinkeel, D.E. Sykes, J.L. Reynolds, B. Bindukumar, A. Adal,
1962–1969.
M. Qi, J. Toh, G. Xu, P.N. Prasad, S.A. Schwartz, Methamphetamine alters
[50]
M.W. Panas, Z. Xie, H.N. Panas, M.C. Hoener, E.J. Vallender, G.M. Miller, Trace
blood brain barrier permeability via the modulation of tight junction
amine associated receptor 1 signaling in activated lymphocytes, J.
expression: implication for HIV-1 neuropathogenesis in the context of drug
Neuroimmune Pharmacol. 7 (4) (2012) 866–876.
abuse, Brain Res. 1203 (2008) 133–148.
[51]
E.A. Reese, J.R. Bunzow, S. Arttamangkul, M.S. Sonders, D.K. Grandy, Trace
[27]
S.H. Ramirez, R. Potula, S. Fan, T. Eidem, A. Papugani, N. Reichenbach, H.
amine-associated receptor 1 displays species-dependent stereoselectivity
Dykstra, B.B. Weksler, I.A. Romero, P.O. Couraud, Y. Persidsky,
for isomers of methamphetamine, amphetamine, and
Methamphetamine disrupts blood-brain barrier function by induction of
para-hydroxyamphetamine, J. Pharmacol. Exp. Ther. 321 (1) (2007)
oxidative stress in brain endothelial cells, J. Cereb. Blood Flow Metab. 29
178–186.
(12) (2009) 1933–1945.
[52]
U. Sriram, B. Haldar, J.M. Cenna, L. Gofman, R. Potula, Methamphetamine
[28]
C. Bemeur, P. Desjardins, R.F. Butterworth, Evidence for
mediates immune dysregulation in a murine model of chronic viral
oxidative/nitrosative stress in the pathogenesis of hepatic encephalopathy,
infection, Front. Microbiol. 6 (2015) 793.
Metab. Brain Dis. 25 (1) (2010) 3–9.
[53]
I.E. Cisneros, A. Ghorpade, Methamphetamine and HIV-1-induced
[29]
R. Rodrigo, S. Erceg, V. Felipo, Neurons exposed to ammonia reproduce the
neurotoxicity: role of trace amine associated receptor 1 cAMP signaling in
differential alteration in nitric oxide modulation of guanylate cyclase in the
astrocytes, Neuropharmacology 85 (2014) 499–507.
cerebellum and cortex of patients with liver cirrhosis, Neurobiol. Dis. 19
[54]
A. Bradaia, G. Trube, H. Stalder, R.D. Norcross, L. Ozmen, J.G. Wettstein, A.
(1–2) (2005) 150–161.
Pinard, D. Buchy, M. Gassmann, M.C. Hoener, B. Bettler, The selective
[30]
N.A. Northrop, L.E. Halpin, B.K. Yamamoto, Peripheral ammonia and blood
antagonist EPPTB reveals TAAR1-mediated regulatory mechanisms in
brain barrier structure and function after methamphetamine,
dopaminergic neurons of the mesolimbic system, Proc. Natl. Acad. Sci. U. S.
Neuropharmacology 107 (2016) 18–26.
A. 106 (47) (2009) 20081–20086.
[31]
T. Martins, S. Baptista, J. Goncalves, E. Leal, N. Milhazes, F. Borges, C.F.
[55]
D. Leo, L. Mus, S. Espinoza, M.C. Hoener, T.D. Sotnikova, R.R. Gainetdinov,
Ribeiro, O. Quintela, E. Lendoiro, M. Lopez-Rivadulla, A.F. Ambrosio, A.P.
Taar1-mediated modulation of presynaptic dopaminergic
Silva, Methamphetamine transiently increases the blood-brain barrier
neurotransmission: role of D2 dopamine autoreceptors,
permeability in the hippocampus: role of tight junction proteins and matrix
Neuropharmacology 81 (2014) 283–291.
metalloproteinase-9, Brain Res. 1411 (2011) 28–40.
[56]
F.G. Revel, J.L. Moreau, R.R. Gainetdinov, A. Bradaia, T.D. Sotnikova, R. Mory,
[32]
N.U. Parikh, R. Aalinkeel, J.L. Reynolds, B.B. Nair, D.E. Sykes, M.J. Mammen,
S. Durkin, K.G. Zbinden, R. Norcross, C.A. Meyer, V. Metzler, S. Chaboz, L.
S.A. Schwartz, S.D. Mahajan, Galectin-1 suppresses methamphetamine
Ozmen, G. Trube, B. Pouzet, B. Bettler, M.G. Caron, J.G. Wettstein, M.C.
induced neuroinflammation in human brain microvascular endothelial
Hoener, TAAR1 activation modulates monoaminergic neurotransmission,
cells: neuroprotective role in maintaining blood brain barrier integrity,
preventing hyperdopaminergic and hypoglutamatergic activity, Proc. Natl.
Brain Res. 1624 (2015) 175–187.
Acad. Sci. U. S. A. 108 (20) (2011) 8485–8490.
[33]
D.E. Rusyniak, Neurologic manifestations of chronic methamphetamine
[57]
L. Jing, J.X. Li, Trace amine-associated receptor 1: a promising target for the
abuse, Neurol. Clin. 29 (3) (2011) 641–655.
treatment of psychostimulant addiction, Eur. J. Pharmacol. 761 (2015)
[34]
NIH, DrugFacts: Methamphetamine, NIH, 2016.
345–352.
[35]
R.R. Metzger, H.M. Haughey, D.G. Wilkins, J.W. Gibb, G.R. Hanson, A.E.
[58]
L. Jing, Y. Zhang, J.X. Li, Effects of the trace amine associated receptor 1
Fleckenstein, Methamphetamine-induced rapid decrease in dopamine
agonist RO5263397 on abuse-related behavioral indices of
transporter function: role of dopamine and hyperthermia, J. Pharmacol. Exp.
methamphetamine in rats, Int. J. Neuropsychopharmacol. 18 (4) (2015).
Ther. 295 (3) (2000) 1077–1085.
[59]
R. Cotter, Y. Pei, L. Mus, A. Harmeier, R.R. Gainetdinov, M.C. Hoener, J.J.
[36]
S.J. Hong, D. Zhang, L.H. Zhang, P. Yang, J. Wan, Y. Yu, T.H. Wang, Z.T. Feng,
Canales, The trace amine-associated receptor 1 modulates
L.H. Li, D.T. Yew, Expression of dopamine transporter in the different
methamphetamine’s neurochemical and behavioral effects, Front. Neurosci.
cerebral regions of methamphetamine-dependent rats, Hum. Exp. Toxicol.
9 (2015) 39.
34 (7) (2015) 707–717.
[60]
E.K. Sloan, J.P. Capitanio, S.W. Cole, Stress-induced remodeling of lymphoid
[37]
J. Yuan, R. Lv, J. Robert Brasic, M. Han, X. Liu, Y. Wang, G. Zhang, C. Liu, Y. Li,
innervation, Brain Behav. Immun. 22 (1) (2008) 15–21.
Y. Deng, Dopamine transporter dysfunction in Han Chinese people with
[61]
R.H. Straub, R. Wiest, U.G. Strauch, P. Harle, J. Scholmerich, The role of the
chronic methamphetamine dependence after a short-term abstinence,
sympathetic nervous system in intestinal inflammation, Gut 55 (11) (2006)
Psychiatry Res. 221 (1) (2014) 92–96.
1640–1649.
[38]
H.M. Haughey, A.E. Fleckenstein, R.R. Metzger, G.R. Hanson, The effects of
[62]
J.D. Johnson, J. Campisi, C.M. Sharkey, S.L. Kennedy, M. Nickerson, B.N.
methamphetamine on serotonin transporter activity: role of dopamine and
Greenwood, M. Fleshner, Catecholamines mediate stress-induced increases
hyperthermia, J. Neurochem. 75 (4) (2000) 1608–1617.
in peripheral and central inflammatory cytokines, Neuroscience 135 (4)
[39]
C. Sogawa, N. Sogawa, J. Tagawa, A. Fujino, K. Ohyama, M. Asanuma, M.
(2005) 1295–1307.
Funada, S. Kitayama, 5-Methoxy-N,N-diisopropyltryptamine (Foxy), a
[63]
L.V. Borovikova, S. Ivanova, M. Zhang, H. Yang, G.I. Botchkina, L.R. Watkins,
selective and high affinity inhibitor of serotonin transporter, Toxicol. Lett.
H. Wang, N. Abumrad, J.W. Eaton, K.J. Tracey, Vagus nerve stimulation
170 (1) (2007) 75–82.
attenuates the systemic inflammatory response to endotoxin, Nature 405
[40]
W.A. Cass, M.W. Manning, Recovery of presynaptic dopaminergic
(6785) (2000) 458–462.
functioning in rats treated with neurotoxic doses of methamphetamine, J.
[64]
D. Martelli, M.J. McKinley, R.M. McAllen, The cholinergic anti-inflammatory
Neurosci. 19 (17) (1999) 7653–7660.
pathway: a critical review, Auton. Neurosci. 182 (2014) 65–69.
[41]
P.O. Allard, J. Rinne, J.O. Marcusson, Dopamine uptake sites in Parkinson’s
[65]
O.V. Pavlov, S.A. Sel’kov, D.V. Lalayan, O.N. Arzhanova, Secretion of
disease and in dementia of the Alzheimer type, Brain Res. 637 (1–2) (1994)
proinflammatory cytokines by villous chorion tissue in spontaneous
262–266.
abortion, Bull. Exp. Biol. Med. 135 (4) (2003) 377–379.
[42]
D.G. Morgan, P.C. May, C.E. Finch, Dopamine and serotonin systems in
[66]
B.L. Bonaz, C.N. Bernstein, Brain-gut interactions in inflammatory bowel
human and rodent brain: effects of age and neurodegenerative disease, J.
disease, Gastroenterology 144 (1) (2013) 36–49.
Am. Geriatr. Soc. 35 (4) (1987) 334–345.
[67]
G. Matteoli, G.E. Boeckxstaens, The vagal innervation of the gut and immune
homeostasis, Gut 62 (8) (2013) 1214–1222.
M.D. Prakash et al. / Pharmacological Research 120 (2017) 60–67
67
[68]
G. Matteoli, P.J. Gomez-Pinilla, A. Nemethova, M. Di Giovangiulio, C. Cailotto,
[94]
A. Burns, P. Ciborowski, Acute exposure to methamphetamine alters
S.H. van Bree, K. Michel, K.J. Tracey, M. Schemann, W. Boesmans, P. Vanden
TLR9-mediated cytokine expression in human macrophage, Immunobiology
Berghe, G.E.A. Boeckxstaens, distinct vagal anti-inflammatory pathway
221 (2) (2016) 199–207.
modulates intestinal muscularis resident macrophages independent of the
[95]
Z. Talloczy, J. Martinez, D. Joset, Y. Ray, A. Gacser, S. Toussi, N. Mizushima,
spleen, Gut 63 (6) (2014) 938–948.
J.D. Nosanchuk, H. Goldstein, J. Loike, D. Sulzer, L. Santambrogio,
[69]
R.D. Herr, E.M. Caravati, Acute transient ischemic colitis after oral
Methamphetamine inhibits antigen processing, presentation, and
methamphetamine ingestion, Am. J. Emerg. Med. 9 (4) (1991) 406–409.
phagocytosis, PLoS Pathog. 4 (2) (2008) e28.
[70]
T.A. Brannan, S. Soundararajan, B.L. Houghton,
[96]
R.V. House, P.T. Thomas, H.N. Bhargava, Comparison of immune functional
Methamphetamine-associated shock with intestinal infarction,
parameters following in vitro exposure to natural and synthetic
MedGenMed 6 (4) (2004) 6.
amphetamines, Immunopharmacol. Immunotoxicol. 16 (1) (1994) 1–21.
[71]
T.L. Carlson, T.P. Plackett, R.A. Gagliano Jr., R.R. Smith,
[97]
S.W. In, E.W. Son, D.K. Rhee, S. Pyo, Methamphetamine administration
Methamphetamine-induced paralytic ileus, Hawaii J. Med. Public Health 71
produces immunomodulation in mice, J. Toxicol. Environ. Health A 68
(2) (2012) 44–45.
(23–24) (2005) 2133–2145.
[72]
T. Suzuki, Regulation of intestinal epithelial permeability by tight junctions,
[98]
N.P. Whitney, T.M. Eidem, H. Peng, Y. Huang, J.C. Zheng, Inflammation
Cell. Mol. Life Sci. 70 (4) (2013) 631–659.
mediates varying effects in neurogenesis: relevance to the pathogenesis of
[73]
N.A. Northrop, B.K. Yamamoto, Methamphetamine effects on blood-brain
brain injury and neurodegenerative disorders, J. Neurochem. 108 (6) (2009)
barrier structure and function, Front. Neurosci. 9 (2015) 69.
1343–1359.
[74]
M. Lyte, L. Vulchanova, D.R. Brown, Stress at the intestinal surface:
[99]
S.D. Mahajan, Z. Hu, J.L. Reynolds, R. Aalinkeel, S.A. Schwartz, M.P. Nair,
catecholamines and mucosa-bacteria interactions, Cell Tissue Res. 343 (1)
Methamphetamine modulates gene expression patterns in monocyte
(2011) 23–32.
derived mature dendritic cells: implications for HIV-1 pathogenesis, Mol.
[75]
P. Bercik, E. Denou, J. Collins, W. Jackson, J. Lu, J. Jury, Y. Deng, P.
Diagn. Ther. 10 (4) (2006) 257–269.
Blennerhassett, J. Macri, K.D. McCoy, E.F. Verdu, S.M. Collins, The intestinal
[100]
K. Golembiowska, J. Konieczny, S. Wolfarth, K. Ossowska, Neuroprotective
microbiota affect central levels of brain-derived neurotropic factor and
action of MPEP, a selective mGluR5 antagonist, in
behavior in mice, Gastroenterology 141 (2) (2011) 599–609, 609. e591-593.
methamphetamine-induced dopaminergic neurotoxicity is associated with
[76]
A. Dive, F. Foret, J. Jamart, P. Bulpa, E. Installe, Effect of dopamine on
a decrease in dopamine outflow and inhibition of hyperthermia in rats,
gastrointestinal motility during critical illness, Intens. Care Med. 26 (7)
Neuropharmacology 45 (4) (2003) 484–492.
(2000) 901–907.
[101]
A. Shah, P.S. Silverstein, S. Kumar, D.P. Singh, A. Kumar, Synergistic
[77]
M.W. Dunser, W.R. Hasibeder, Sympathetic overstimulation during critical
cooperation between methamphetamine and HIV-1 gsp120 through the
illness: adverse effects of adrenergic stress, J. Intens. Care Med. 24 (5) (2009)
P13K/Akt pathway induces IL-6 but not IL-8 expression in astrocytes, PLoS
293–316.
One 7 (12) (2012) e52060.
[78]
M.G. Zizzo, F. Mule, M. Mastropaolo, R. Serio, D1 receptors play a major role
[102]
Y. Zhang, X. Lv, Y. Bai, X. Zhu, X. Wu, J. Chao, M. Duan, S. Buch, L. Chen, H.
in the dopamine modulation of mouse ileum contractility, Pharmacol. Res.
Yao, Involvement of sigma-1 receptor in astrocyte activation induced by
61 (5) (2010) 371–378.
methamphetamine via up-regulation of its own expression, J.
[79]
M. Carabotti, A. Scirocco, M.A. Maselli, C. Severi, The gut-brain axis:
Neuroinflamm. 12 (2015) 29.
interactions between enteric microbiota, central and enteric nervous
[103]
H. Yang, H.S. Hreggvidsdottir, K. Palmblad, H. Wang, M. Ochani, J. Li, B. Lu, S.
systems, Ann. Gastroenterol. 28 (2) (2015) 203–209.
Chavan, M. Rosas-Ballina, Y. Al-Abed, S. Akira, A. Bierhaus, H.
[80]
S.O. Fetissov, P. Dechelotte, The new link between gut-brain axis and
Erlandsson-Harris, U. Andersson, K.J. Tracey, A critical cysteine is required
neuropsychiatric disorders, Curr. Opin. Clin. Nutr. Metab. Care 14 (5) (2011)
for HMGB1 binding to Toll-like receptor 4 and activation of macrophage
477–482.
cytokine release, Proc. Natl. Acad. Sci. U. S. A. 107 (26) (2010) 11942–11947.
[81]
E.A. Mayer, K. Tillisch, A. Gupta, Gut/brain axis and the microbiota, J. Clin.
[104]
M. Bustin, Regulation of DNA-dependent activities by the functional motifs
Invest. 125 (3) (2015) 926–938.
of the high-mobility-group chromosomal proteins, Mol. Cell. Biol. 19 (8)
[82]
A. Louveau, I. Smirnov, T.J. Keyes, J.D. Eccles, S.J. Rouhani, J.D. Peske, N.C.
(1999) 5237–5246.
Derecki, D. Castle, J.W. Mandell, K.S. Lee, T.H. Harris, J. Kipnis, Structural and
[105]
J.R. Klune, R. Dhupar, J. Cardinal, T.R. Billiar, A. Tsung, HMGB1: endogenous
functional features of central nervous system lymphatic vessels, Nature 523
danger signaling, Mol. Med. 14 (7–8) (2008) 476–484.
(7560) (2015) 337–341.
[106]
M.S. Quinton, B.K. Yamamoto, Neurotoxic effects of chronic restraint stress
[83]
A.I. Petra, S. Panagiotidou, E. Hatziagelaki, J.M. Stewart, P. Conti, T.C.
in the striatum of methamphetamine-exposed rats, Psychopharmacology
Theoharides, Gut-microbiota-brain axis and its effect on neuropsychiatric
(Berl.) 193 (3) (2007) 341–350.
disorders with suspected immune dysregulation, Clin. Ther. 37 (5) (2015)
[107]
J. Raudensky, B.K. Yamamoto, Effects of chronic unpredictable stress and
984–995.
methamphetamine on hippocampal glutamate function, Brain Res. 1135 (1)
[84]
D. Kerr, C. Krishnan, M.L. Pucak, J. Carmen, The immune system and
(2007) 129–135.
neuropsychiatric diseases, Int. Rev. Psychiatry (Abingdon, England) 17 (6)
[108]
J.L. Cadet, S. Jayanthi, X. Deng, Speed kills: cellular and molecular bases of
(2005) 443–449.
methamphetamine-induced nerve terminal degeneration and neuronal
[85]
D.E. Rusyniak, Neurologic manifestations of chronic methamphetamine
apoptosis, FASEB J. 17 (13) (2003) 1775–1788.
abuse, Psychiatr. Clin. North Am. 36 (2) (2013) 261–275.
[109]
T.R. Guilarte, M.K. Nihei, J.L. McGlothan, A.S. Howard,
[86]
R. Harms, B. Morsey, C.W. Boyer, H.S. Fox, N. Sarvetnick, Methamphetamine
Methamphetamine-induced deficits of brain monoaminergic neuronal
administration targets multiple immune subsets and induces phenotypic
markers: distal axotomy or neuronal plasticity, Neuroscience 122 (2) (2003)
alterations suggestive of immunosuppression, PLoS One 7 (12) (2012)
499–513.
e49897.
[110]
Y.-M. Lin, W.-W. Sung, M.-J. Hsieh, S.-C. Tsai, H.-W. Lai, S.-M. Yang, K.-H.
[87]
M. Saito, T. Yamaguchi, T. Kawata, H. Ito, T. Kanai, M. Terada, M. Yokosuka,
Shen, M.-K. Chen, H. Lee, K.-T. Yeh, C.-J. Chen, High PD-L1 expression
T.R. Saito, Effects of methamphetamine on cortisone concentration, NK cell
correlates with metastasis and poor prognosis in oral squamous cell
activity and mitogen response of T-lymphocytes in female cynomolgus
carcinoma, PLoS One 10 (11) (2015) e0142656.
monkeys, Exp. Anim. 55 (5) (2006) 477–481.
[111]
J. Kiyasu, H. Miyoshi, A. Hirata, F. Arakawa, A. Ichikawa, D. Niino, Y. Sugita, Y.
[88]
H. Peerzada, J.A. Gandhi, A.J. Guimaraes, J.D. Nosanchuk, L.R. Martinez,
Yufu, I. Choi, Y. Abe, N. Uike, K. Nagafuji, T. Okamura, K. Akashi, R.
Methamphetamine administration modifies leukocyte proliferation and
Takayanagi, M. Shiratsuchi, K. Ohshima, Expression of programmed cell
cytokine production in murine tissues, Immunobiology 218 (8) (2013)
death ligand 1 is associated with poor overall survival in patients with
1063–1068.
diffuse large B-cell lymphoma, Blood 126 (19) (2015) 2193–2201.
[89]
H. Liang, X. Wang, H. Chen, L. Song, L. Ye, S.H. Wang, Y.J. Wang, L. Zhou, W.Z.
[112]
J. He, Y. Hu, M. Hu, B. Li, Development of PD-1/PD-L1 pathway in tumor
Ho, Methamphetamine enhances HIV infection of macrophages, Am. J.
immune microenvironment and treatment for non-small cell lung cancer,
Pathol. 172 (6) (2008) 1617–1624.
Sci. Rep. 5 (2015) 13110.
[90]
Q. Yu, D. Zhang, M. Walston, J. Zhang, Y. Liu, R.R. Watson, Chronic
[113]
V. Mishra, H. Schuetz, J. Haorah, Differential induction of PD-1/PD-L1 in
methamphetamine exposure alters immune function in normal and
neuroimmune cells by drug of abuse, Int. J. Physiol. Pathophysiol.
retrovirus-infected mice, Int. Immunopharmacol. 2 (7) (2002) 951–962.
Pharmacol. 7 (2) (2015) 87–97.
[91]
J.M. Loftis, A. Janowsky, Neuroimmune basis of methamphetamine toxicity,
[114]
K.A. Sheppard, L.J. Fitz, J.M. Lee, C. Benander, J.A. George, J. Wooters, Y. Qiu,
Int. Rev. Neurobiol. 118 (2014) 165–197.
J.M. Jussif, L.L. Carter, C.R. Wood, D. Chaudhary, PD-1 inhibits T-cell receptor
[92]
A. Shah, P.S. Silverstein, D.P. Singh, A. Kumar, Involvement of metabotropic
induced phosphorylation of the ZAP70/CD3zeta signalosome and
glutamate receptor 5, AKT/PI3K signaling and NF-kappaB pathway in
downstream signaling to PKCtheta, FEBS Lett. 574 (1–3) (2004) 37–41.
methamphetamine-mediated increase in IL-6 and IL-8 expression in
astrocytes, J. Neuroinflamm. 9 (2012) 52.
[93]
J.A. Najera, E.A. Bustamante, N. Bortell, B. Morsey, H.S. Fox, T. Ravasi, M.C.
Marcondes, Methamphetamine abuse affects gene expression in
brain-derived microglia of SIV-infected macaques to enhance inflammation
and promote virus targets, BMC. Immunol. 17 (1) (2016) 7.
Document Outline